Global warming: it’s here, we did it, we can fix it.
In 2007, the United Nations-based Intergovernmental Panel on Climate Change confirmed that human-induced global warming is real, and that the costs could be dire: increased heat waves, floods, drought, famine and disease may spell the deaths of millions.
Why, then, do recent Gallup polls show the number of Americans who acknowledge the seriousness of global warming decreasing from 66 percent to 51 percent between 2008 and 2011?
Perhaps the climate-change issue has become so complex and so inadequately conveyed that the public has forgotten, or maybe never really understood, what is at stake.
As one of the gravest challenges facing our society today, a basic understanding of what climate change is and what it is not is absolutely essential.
Climate change refers to long-term changes in the chemical make-up of the atmosphere and its impact on the environment and life. For instance, after the Earth formed about 4.5 billion years ago, the atmosphere consisted of toxic gases like methane and carbon dioxide.However, once microorganisms began producing oxygen about 2.5 billion years ago, oxygen started to become prevalent in the atmosphere.
Increasing levels of oxygen spurred the evolution of oxygen-dependent life and helped create the ozone layer, which shields us from the sun's harmful UV radiation.
When discussing present-day climate change, the most important gases are the greenhouse gases, such as carbon dioxide, methane, and water vapor, which have the ability to trap heat from the sun.
Global warming occurs when these gases build up in the atmosphere.
The atmospheric abundance of a gas can be measured in parts per million (ppm).
This means that, in a given sample of 1 million air molecules, a certain number will be, say, carbon dioxide.
In 1958, atmospheric carbon dioxide levels were at 315 ppm, and by 2010 had reached 390 ppm. Never in the past 650,000 years has carbon dioxide reached such a level.
If more greenhouse gases like carbon dioxide accumulate in the atmosphere, global warming will ensue.
Climate change in the form of global warming is immediately relevant to the current and future welfare of our species: Rising sea levels as a result of melting ice caps will inundate coastal communities, drought-induced famine will devastate Africa, and tropical rain forests will give way to savannah grasslands.Closer to home, decreased snowpack in the Sierra Nevada mountains may reduce California's water supplies, and Southern California, in particular, will likely experience more frequent, more intense heat waves.
While the Earth has experienced global warming before, it has usually occurred over long spans of time (hundreds of thousands to millions of years, not decades).
If we are to face the urgency of present-day climate change, we must understand the basic science behind the issue.
"There is a wide gap between what is understood about global climate change by the relevant scientific community and what is known by policymakers and the public," says James Hansen, director of the NASA Goddard Institute for Space Studies in New York and one of the world's leading climate scientists.
Along these lines, a new partnership between UC Riverside and NASA, called "Down to Earth Climate Science," will help educate local high school and university students on how to scientifically observe the Earth's climate using NASA-based climate change data and modeling tools.
The overall goal of the collaboration is to give students -- tomorrow's political and scientific leaders -- a firm background in climate-change science and an ability to effectively communicate what they learn to their peers.
"Down to Earth Climate Science" will also give students within the Riverside Unified School District the chance to work one-on-one with a UCR scientist to develop a science-fair project centered around a specific-climate change issue.
At a meeting last month, several RUSD middle school and high school students got to meet with potential UCR mentors to discuss science-fair-project ideas. From measuring energy consumption patterns at home to studying long-term snowpack trends in the Sierras, students can transform original insights into climate-related solutions.
Armed with a basic understanding of climate change science, we have choices. We can put the paper down, ignore climate change, perhaps turn on the TV and continue on as before.Or we can take action as a community, country and as citizens of the world.
To start, we can engage in a conversation that begins by acknowledging that we have changed, and are changing, the chemical composition of our atmosphere, the costs of which may be more than our species can bear.
This article appeared in the opinion section of the Press-Enterprise newspaper.
Oxygen-free Early Oceans Likely Delayed Rise of Life on Planet
New findings illustrate how earliest life on Earth co-evolved with oceanic chemistry
RIVERSIDE, Calif. – Geologists at the University of California, Riverside have found chemical evidence in 2.6-billion-year-old rocks that indicates that Earth’s ancient oceans were oxygen-free and, surprisingly, contained abundant hydrogen sulfide in some areas.
“We are the first to show that ample hydrogen sulfide in the ocean was possible this early in Earth’s history,” said Timothy Lyons, a professor of biogeochemistry and the senior investigator in the study, which appears in the February issue of Geology. “This surprising finding adds to growing evidence showing that ancient ocean chemistry was far more complex than previously imagined and likely influenced life’s evolution on Earth in unexpected ways – such as, by delaying the appearance and proliferation of some key groups of organisms.”Spotlight: Tectonics and the Recent Haitian and Chilean Earthquakes
The Earth is layered. The layer we play and dance on, the crust, is the outermost layer. Beneath the crust is the honey-like mantle and under that is the liquid outer-core. The final ‘layer,’ the core, is a solid sphere. From where you’re standing/playing/dancing to the center of the core is a bit under 4,000 miles (to give a sense of size, the deepest hole humans have ever drilled is only 7.5 miles deep). Of all the layers, the mantle is the most massive, comprising more than 80% of the entire mass of the planet.
The layering of the Earth that is most relevant to earthquakes is called the lithosphere, of which consists of the crust and the upper mantle. The lithosphere is broken up into tectonic plates. There about 12 major plates on our planet. Earthquakes like the ones that rocked Haiti and Chile occur at the boundaries of these plates – they are the consequence of adjacent plates moving relative to one another, and they often release astronomical amounts of stress in the form of quakes that can, as we saw in the news, devastate entire nations. Plate boundaries take on three main forms: they are either spreading apart (also called a divergent boundary), sliding past each other (also known as a transform fault boundary, like the San Andreas), or they’re colliding into one another (this is called a convergent boundary). Collectively, all this plates-smacking-into-plates business is called plate tectonics, and its discovery is long and storied. Let’s take a look:
Alfred Wegener – a German geoscientist with a stalwart will who died on his way back from a rescue operation to bring supplies to a remote research station in the middle of the Greenland icecap – correctly noted how well the coastlines of Africa and South America appear to fit together like two pieces of a puzzle. While he wasn’t the first person to notice this peculiarity, Wegener was the first to use rock and fossil evidence to prove without a doubt that the Americas were once connected to Africa and Europe. Ultimately this led Wegener to propose his now-famous and now-confirmed theory of continental drift, which basically states that the continents of the world are in motion relative to one another rather than being static. Wegener’s theory meant that Charles Schuchert, a 19th and 20th century paleontologist who said that “the striking similarity of the coastlines of Africa and Brazil must have been made by Satan,” could finally rest easy knowing that the Desolate One probably had nothing to do with the geographic layout of the Earth’s continents.
While Wegener presented good evidence to show that the Earth’s continents are in motion, his theory was missing one key part: a mechanism. Sure you found two of the same fossil on two separate continents, proving that they were once connected, but how, Dr. Wegener, do the continents move? Wegener had no good answer for this, and it wasn’t until World War II that evidence for a mechanism for continental drift would begin accumulating.
Enter Professor Harry Hammond Hess, who was a naval officer during World War II who performed echo-sounding surveys of the Pacific Ocean’s seafloor while his warship was traveling to and from various battles and skirmishes. These echo surveys, which provided an elevation map of the Pacific’s seafloor, gave Hess the data he needed to come up with his essential theory of seafloor spreading which, simply put, is exactly what it sounds like: mantle magma shoots up from underneath the crust (continental or oceanic), forming what’s called a spreading center (or a divergent tectonic plate boundary, aka a mid-ocean ridge system) that pushes the crust out and away and into other plates, which in turn can form new plate boundaries which can become ridden with stress. In Photo 1, these spreading centers are found in the red zones of the map (red = new ‘hot’ crust, while the ‘colder’ colors like green and blue = older crust that’s being pushed away by the red). It's this pushing and prodding of the plates against other plates that causes their movement which, in turn, causes earthquakes like the ones in Haiti, Chile and in any other area on the planet that rests at the boundary between two or three (but never four) different tectonic plates. Note in Photo 2 how both Haiti and Chile are found on plate boundaries. Coincidence? Haha, yeah, sure.
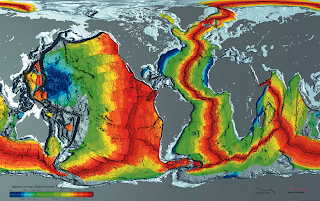
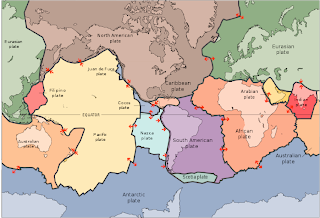
QA #3
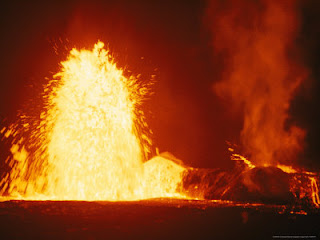
QA #2
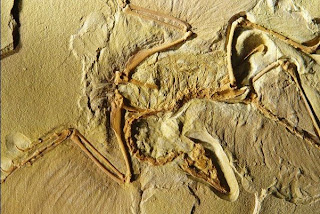
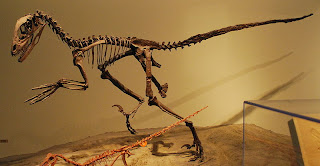
Similarities between Archaeopteryx and Deinonychus include three-toed feet, sharp-toothed jaws, wishbones, and a long, bony tail. Archaeopteryx also bore claws on its very-reduced forelimb fingers – something no bird alive today can boast. On the flip side, there have been quite a few theropod fossils discovered that have bird-like characteristics, the most notable being feathers; the first dinosaur fossil found with feathers (or feather impressions, to be exact) is that of Sinosauropteryx (pronounced SIGN-o-sar-OPT-er-ix).
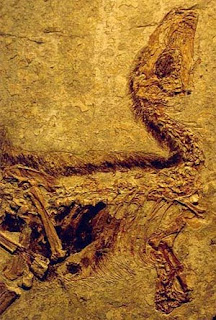
Photo 3: Sinosaurpteryx fossil from China.
This 140 million-year-old dinosaur, which measures in at less than half a foot tall and about two feet long, was discovered in the 1990’s in China’s Liaoning province (an unusually fossil-rich region) by full-time farmer part-time fossil hunter Li Yinfang. As you might be able to tell from its picture, Sinosauropteryx was endowed with small yet entirely respectable feathers: sweet! Now, between Archaeopteryx,
Anyhow, there you have it, folks. While there are a million more words to be said about the birds-are-dinosaurs topic, I think I’ll let you digest the small morsels given above. You can also now relish in the fact that the next time someone points at a bird and says “I think I read somewhere that birds are dinosaurs,” you’ll be able to say “yes, that’s 100% correct. Birds are non-avian dinosaurs derived from theropods,” to which they’ll probably reply “oh, snap!”
Next up: QA #3 How much lava, exactly, erupts from an exploding volcano? Is there much variability?
Spotlight: Mount Diablo, California
Mount Diablo is located roughly thirty miles east of San Francisco in Contra Costa County, California. The mountain’s summit, standing at about 3,848 feet above sea level, offers a striking view of the San Francisco bay area to the west and California’s Central Valley to the east (on the occasional clear day the sentinel-like Sierra Nevada mountain range that looms over 100 miles off to the east is also visible). The rocks that make up the summit, and which make this 360-degree view possible, consist mostly of greenstone (an originally volcanic rock that has been metamorphosed, or squished around) and sedimentary rocks that include sandstone, shale, and red, microfossil-rich chert (microfossils, in this case, refer to radiolarians - very, very, very tiny amoeba that produce sometimes-dazzling mineral skeletons). All of these rocks originated in a submarine environment well over 90 million years ago, since when they have undergone an incredible amount of disfiguration and uplift via tectonics (BOOM!! CRASH! ShaZiNG!! is what tectonics sounds like J).
If the rocks described above formed over 90 million years, does this mean that Mount Diablo itself is also that old? No, not necessarily. A nearby bed of volcanic ash that the mountain punched through as it uplifted is about 4.8 million years old; this fact allows us to deduce that the mountain itself must be 4.8 million years old or younger (we know this because, if the bed of ash were younger than Mount Diablo, it would have had to be deposited over and on top of the mountain, which it clearly isn’t).
Hope you learned something new about a very special mountain in California! Up next: QA #2: Are Birds Really Dinosaurs??
See you!
QA #1
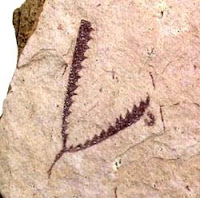
Q How does a dead organism become a fossil?
A Most organisms do not become fossils after they die. This is because the conditions that promote fossilization are very rare. Also, because hard parts like shells and bones have a better chance of getting preserved than soft parts like skin and cartilage, preservation is not equal among all organisms. When fossilization does occur, however, the process can take many forms.
Some organisms can be preserved with their soft tissues almost completely unaltered; one famous example are the woolly mammoths that were found frozen in a lifelike state in the Siberian tundra in 1901. Permineralization, another method of fossilization, occurs when water-borne minerals like calcium carbonate flow through and precipitate out within porous tissues like bone and wood. Recrystalization, on the other hand, happens when the original mineral components of an organism alter into chemically different, though texturally similar crystals. If a fossil becomes filled with sediment and then dissolves away, it can leave behind an internal mold, or steinkern. Other fossils, like those of plants and graptolites (see picture), can be preserved as a thin film of usually dark carbon within a rock.
The activity of an organism can also get preserved in the rock record. These so-called trace fossils, or ichnofossils, can reflect what the organism was doing while it was alive, what it was eating, or what kind of an environment it lived in. Examples include preserved footprints, burrows, fecal matter (or coprolites), and root casts from an ancient plant.